Posted on December 13, 2023 in ASRC News, Photonics Initiative
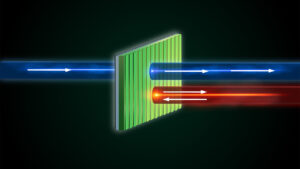
Using a thin layer of nano-patterned silicon, scientists are able to transmit light unidirectionally and prevent incoming reflections, all without the use of magnets.
NEW YORK, December 8, 2023 — Much like the ebb and flow of ocean waves, light and sound waves bounce off objects and reflect back to their source. This inherent symmetry is known as reciprocity, and it can be problematic for the operation of many devices. For example, reflected waves from a powerful laser source can interfere with or even damage the device transmitting the signal.
Special magnets, routers, and isolators, which are bulky and expensive, are normally needed to achieve asymmetrical wave propagation and overcome such problems. But a recently published study in Nature Photonics details a successful experiment conducted by a multi-institutional team of researchers that allowed them to break wave reciprocity without use of those devices.
The work was led by Andrea Alù, founding director of the Photonics Initiative at the Advanced Science Research Center at the CUNY Graduate Center and distinguished professor of physics at the Graduate Center, and conducted in collaboration with colleagues at AMOLF in the Netherlands and Wayne State University. The team’s goal was to find a solution that could break light symmetry in strong laser beams propagating in free space without resorting to the use of magnetic materials, which often face equipment integration challenges. The approach focused on combining geometrical asymmetries with optical nonlinearities. These properties arise in materials that change their optical responses based on the intensity of the light flowing through them. Interestingly, the researchers found that the desired asymmetry could be achieved within a simple design and by using common materials. Their demonstration utilizes an ultrathin layer of silicon patterned at the nanoscale.
“Silicon is the most common material you can think of in the context of optical technologies, and it is already the dominant material in so many of our devices and technologies,” said Alù. “We nanostructured a thin silicon film, only 100 nanometers in thickness or so, and demonstrated that, with the right shape and structure, we can largely enhance its optical non-linearity and leverage it to break reciprocity for light propagation.”
The researchers started from a flat layer of silicon and etched lines onto it to achieve a sharp spectral response that helped enhance the otherwise weak nonlinearity of silicon. Crucially, the bottom part of the thin layer was left unetched in order to break the geometrical symmetry by just the right amount. Such geometrical asymmetry, combined with the enhanced nonlinearity, allows light to pass through when the film is hit from one side. Yet, when the patterned film is hit from the opposite side, the light is reflected away. In order to achieve this fit, the researchers engineered a “quasi-bound state in the continuum” in the device. Similar to an optical cavity made out of two mirrors, this state allows for light to be trapped in the thin engineered silicon film, enhancing its nonlinearity and intensity in a controllable fashion.
“Our design is all about simplicity,” said Michele Cotrufo, assistant professor at the University of Rochester who was previously a postdoctoral researcher in Alù’s lab. “The idea of breaking reciprocity with tailored nonlinear materials has been floating around for some time, but no one was able to experimentally demonstrate it for light waves traveling in free space. We asked ourselves what the simplest, most practical design that still has all the right ingredients for the idea to work. Those ingredients for us were the patterning of a simple silicon film to create the required frequency dependence, vertical asymmetry to break mirror symmetry, and in-plane nanostructures to control the optical confinement. When we put these ingredients together, we realized that this was the best viable solution to demonstrate the working principle of this phenomenon.”
While the current model operates within specific light bandwidths, the researchers aspire to create versatile models for different wavelengths, including those beyond optical frequencies. Exploring other nonlinear effects is also on the agenda, as the current material mostly relies on thermal phenomena to alter the refractive index. While these effects arise in a matter of microseconds, the team says it may not be fast enough for certain applications.
Despite its limitations, the demonstrated silicon device holds vast potential applications. Beyond preventing reflections that are harmful to lasers, it may find uses in communication devices, such as cellphones and Lidar (the light equivalent of radar). Lidar is crucial in various technologies, including self-driving cars employing lasers to sense surrounding objects. The researchers aim to expand the reach of their silicon device, paving the way for widespread applications in diverse fields.
The work was supported by the Air Force Office of Scientific Research, the Simons Foundation, and the Netherlands Organization for Scientific Research (NWO).
About the Advanced Science Research Center at the CUNY Graduate Center
The Advanced Science Research Center at the CUNY Graduate Center (CUNY ASRC) is a world-leading center of scientific excellence that elevates STEM inquiry and education at CUNY and beyond. The CUNY ASRC’s research initiatives span five distinctive, but broadly interconnected disciplines: nanoscience, photonics, neuroscience, structural biology, and environmental sciences. The center promotes a collaborative, interdisciplinary research culture where renowned and emerging scientists advance their discoveries using state-of-the-art equipment and cutting-edge core facilities.