Posted on November 2, 2023 in ASRC News, Photonics Initiative
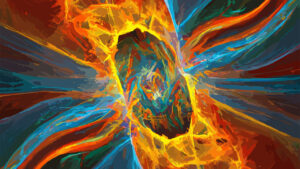
Studying how light beams interfere with each other in a stadium shaped arena gives researchers insight on its complex behavior.
NEW YORK, November 2, 2023 — Harnessing and controlling light is vital for the development of technology, including energy harvesting, computation, communications, and biomedical sensing. Yet, in real-world scenarios, complexity in light’s behavior poses challenges for its efficient control. Physicist Andrea Alù likens the behavior of light in chaotic systems to the initial break shot in a game of billiards.
“In billiards, tiny variations in the way you launch the cue ball will lead to different patterns of the balls bouncing around the table,” said Alù, Einstein Professor of Physics at the CUNY Graduate Center, founding director of the Photonics Initiative at the CUNY Advanced Science Research Center and distinguished professor at CUNY. “Light rays operate in a similar way in a chaotic cavity. It becomes difficult to model to predict what will happen because you could run an experiment many times with similar settings, and you’ll get a different response every time.”
In a new study published in Nature Physics, a team led by researchers at CUNY describe a new platform for controlling the chaotic behavior of light by tailoring its scattering patterns using light itself. The project was led by co-first authors Xuefeng Jiang, a former postdoctoral researcher in Alù’s lab who is now assistant professor of Physics with Seton Hall University, and Shixiong Yin, a graduate student in Alù’s lab.
Conventional platforms for studying light’s behaviors typically use circular or regularly shaped resonant cavities in which light bounces and scatters in more predictable patterns. In a circular cavity, for example, only predictable and distinct frequencies (colors of light) survive, and each supported frequency is associated with a specific spatial pattern, or mode. One mode at a single frequency is sufficient to understand the physics at play in a circular cavity, but this approach does not unleash the full complexity of light behaviors seen in complex platforms, Jaing said.
“In a cavity that supports chaotic patterns of light, any single frequency injected into the cavity can excite thousands of light patterns, which is conventionally thought to doom the chances of controlling the optical response,” Jaing said. “We have demonstrated that it is possible to control this chaotic behavior.”
To address the challenge, the team designed a large stadium-shaped cavity with an open top and two channels on opposing sides that direct light into the cavity. As incoming light scatters off the walls and bounces around, a camera above records the amount of light escaping the stadium and its spatial patterns.
The device features knobs on its sides to manage the light intensity at the two inputs, and the delay between them. Opposing channels cause the light beams to interfere with each other in the stadium cavity, enabling the control of one beam’s scattering by the other through a process known as coherent control—essentially, using light to control light, according to Alù. By adjusting the relative intensity and delay of the light beams entering the two channels, remarkably, researchers consistently altered the light’s radiation pattern outside the cavity.
This control was enabled through a rare behavior of light in resonant cavities, called “reflectionless scattering modes” (RSMs), which had been theoretically predicted before but not observed in optical cavity systems. According to Yin, the ability to manipulate RSMs demonstrated in this work allows for the efficient excitation and control of complex optical systems, which has implications for energy storage, computing, and signal processing.
“We found at certain frequencies our system can support two independen, overlapping RSMs, which cause all of the light to enter the stadium cavity without reflections back to our channel ports, thus enabling its control,” said Yin. “Our demonstration deals with optical signals within the bandwidth of optical fibers that we use in our daily life, so this finding paves a new way for better storage, routing, and control of light signals in complex optical platforms.”
The researchers aim to incorporate additional knobs in future studies, offering more degrees of freedom to unravel further complexities in the behavior of light.
“Coherent Control of Chaotic Optical Microcavity with Reflectionless Scattering Modes,” by Xuefeng Jiang, Shixiong Yin, Huanan Li, Jiamin Quan, Heedong Goh, Michele Cotrufo, Julius Kullig, Jan Wiersig, and Andrea Alù.
About the Graduate Center of The City University of New York
The CUNY Graduate Center is a leader in public graduate education devoted to enhancing the public good through pioneering research, serious learning, and reasoned debate. The Graduate Center offers ambitious students nearly 50 doctoral and master’s programs of the highest caliber, taught by top faculty from throughout CUNY — the nation’s largest urban public university. Through its nearly 40 centers, institutes, initiatives, and the Advanced Science Research Center, the Graduate Center influences public policy and discourse and shapes innovation. The Graduate Center’s extensive public programs make it a home for culture and conversation.
About the Advanced Science Research Center at the CUNY Graduate Center
The Advanced Science Research Center at the CUNY Graduate Center (CUNY ASRC) is a world-leading center of scientific excellence that elevates STEM inquiry and education at CUNY and beyond. The CUNY ASRC’s research initiatives span five distinctive, but broadly interconnected disciplines: nanoscience, photonics, neuroscience, structural biology, and environmental sciences. The center promotes a collaborative, interdisciplinary research culture where renowned and emerging scientists advance their discoveries using state-of-the-art equipment and cutting-edge core facilities.